New theory adds to the black hole debate
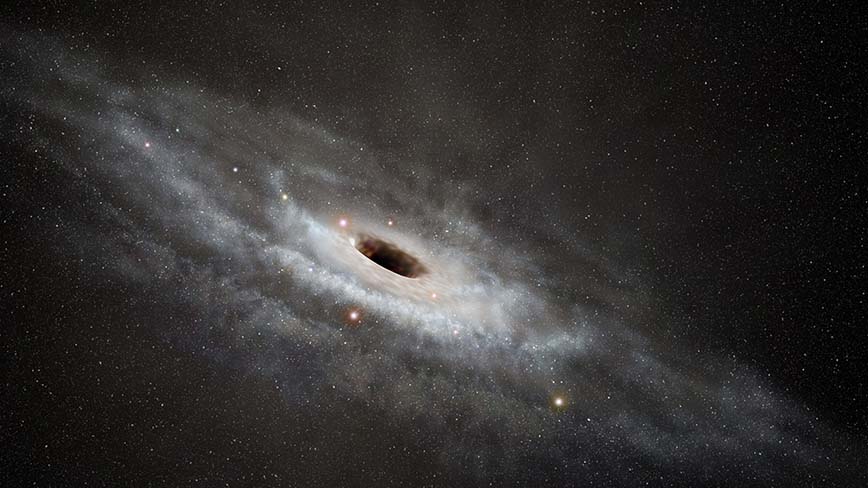
Scientists have long been baffled by the black holes of the universe, the most famous theories created by British physicist Stephen Hawking. Now Erik Aurell, professor at KTH Royal Institute of Technology, and a team of other researchers have developed a new theory of random Gaussian multiphoton states, contributing to the debate.
Scientists know that black holes exist for real from many kinds of observations. For example, there is a black hole with a mass four times that of the Sun about 27,000 light years from us. The closest black hole known to scientists is Gaia BH1, which is 1 560 light years away.
According to a theory by British physicist Stephen Hawking, black holes are not completely black, rather they are dark grey and slowly boiling away. Hawking's theory has kept scientists busy for half a century because it is difficult to combine with quantum mechanics. Hawking himself long argued that the difference is so great that quantum mechanics cannot apply in the interior of black holes, but he changed his mind towards the end of his life and said it should somehow be possible.
Now Erik Aurell, professor at KTH Royal Institute of Technology, together with a team of other researchers, has developed a new theory of random Gaussian multiphoton states, which contributes to the debate and is published in the article ‘Random Pure Gaussian States and Hawking Radiation’.
"We believe that the methods we developed can be applied to other problems as well," says Erik Aurell, Professor of Biological Physics at KTH Royal Institute of Technology.
Aurell and his team's solution is based on the fact that what Hawking first discovered was that his radiation looks maximally disordered if you look at one mode at a time, that is all light that has approximately the same frequency. If summed over all modes, this disorder is difficult to combine with quantum mechanics. If this is possible, the disorder must be an effect of looking at one mode at a time and not all modes at once. In quantum physics, such a phenomenon is called quantum entanglement. The problem is that if all the modes in the Hawking radiation are entangled with each other, then each pair of modes should also be entangled. Hawking and others showed early on that this cannot be the case, at least not for different modes of the Hawking radiation emitted from the black hole at about the same time.
Can contribute
How this is actually the case, nobody knows. But one can ask what it might typically look like. There are many different states of the total Hawking radiation that both do not violate quantum mechanics and are such that they look like Hawking's prediction, mode by mode. Taking a random such state, will the modes then be pairwise entangled with each other or not? The answer that Aurell's team came up with is that they almost certainly won't.
From a methodological perspective, the team's results can be seen as an example of how difficult or perhaps intractable questions can sometimes be made easier by making the problem bigger. The number of modes in the Hawking radiation from a black hole is almost unimaginably large, and it is precisely in that limit, which increases with the mass of the black hole, that the problem becomes solvable. Although the analogy is somewhat long-winded, similar effects, such as combinatorial optimisation problems, also occur in AI.
From a more practical perspective, the research can contribute to new ways of processing quantum entangled photons and find ways to improve sensors, radiation and microscopy.
"Now we will look at a technical follow-up, improvement and simplification of the theory," says Erik Aurell